به خوبی پذیرفته شده است که ذرات سوخت هستهای پوشش داده شده ی TRISO (ایزوتروپ سه ساختاری) دارای قابلیت نگهداری محصولات شکافت تا °C1600 هستند، ولی بالای این دما محصولات شکافت میتوانند از طریق کربن پیرولیتی (PyC) و پوششهای کاربید سیلیسیم نفوذ کنند که قادر میباشند به شکل سدهای محدود کننده در این سوخت عمل کنند. با وجود دههها تحقیق و توسعه، در رابطه با محدودهی دمای واقعی این سوخت، شناخت کمی حاصل شده است. برای درک دمای واقعی این سوخت، پوششهای PyC تولید شده توسط لایه نشانی با بخار شیمیایی بستر سیال در دمای °C1000، °C1400 و °C1700 به مدت 200 ساعت در اتمسفر خنثی حرارت دهی شدند. مشاهده شد که بالای °C1400 انیزوتروپی، اندازه سامان و درجهی گرافیته شدن به دو برابر مقدار واقعی آن افزایش مییابد. به علاوه، در °C1700 بعضی از نمونهها تشکیل نانو تخلخلها را نشان دادند، که میتواند به دلیل واقعی بودن محدودهی دمای حداکثر سوخت یا حداقل، به آن مربوط باشد. نفوذپذیری افزایش یافتهی عناصر به دلیل تغییرات میکروساختاری با توجه به آزمایشهای نفوذ نقره تایید شدهاست. علاوه بر این، مشاهده شد که تمام نمونهها سطح یکسانی از گرافیته شدن را تحمل نمیکنند. به این ترتیب گمان میشود که پوششهای PyC میتوانند قابلیت خود را برای نگهداری محصولات شکافت حتی پس از گردش دمایی بالای °C1600 حفظ کنند.
اثر عملیات حرارتی بر ریزساختار و نفوذ نقره در پوششهای کربنی پیرولیتیک
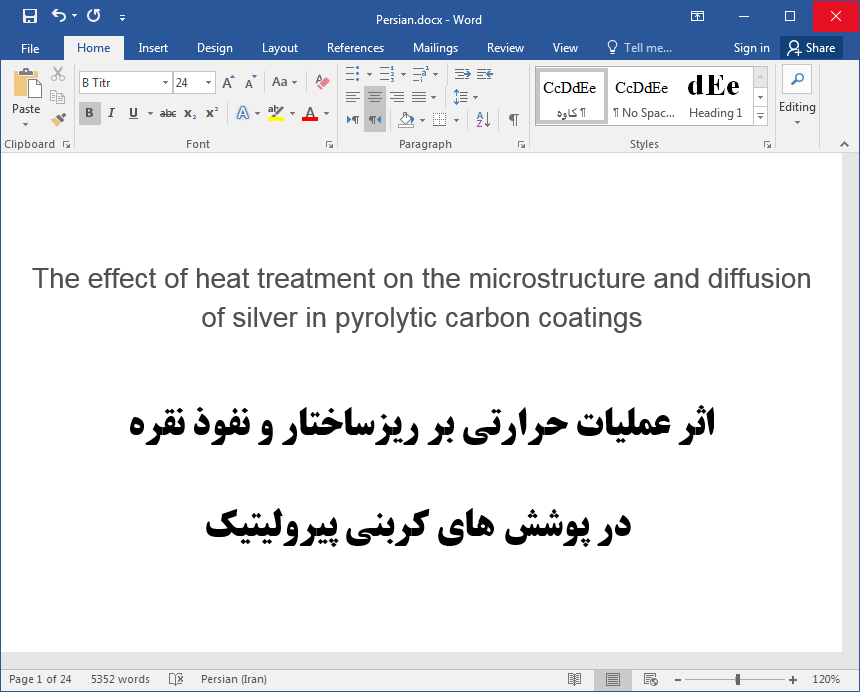
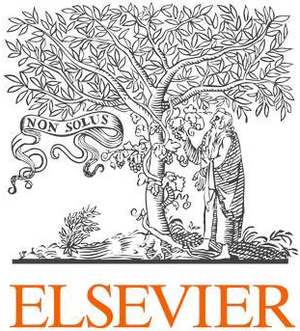
28,700 تومانشناسه فایل: 5258
- حجم فایل ورد: 1.2MB حجم پیدیاف: 933.1KB
- فرمت: فایل Word قابل ویرایش و پرینت (DOCx)
- تعداد صفحات فارسی: 24 انگلیسی: 10
- دانشگاه:Centro de Investigación y de Estudios Avanzados del IPN (CINVESTAV), Unidad Saltillo, Av. Industria Metalúrgica 1062, Ramos Arizpe, 25900, Mexico
- ژورنال: Carbon (1)
چکیده
مقدمه
حادثهی هستهای در فوکوشیما [Fukushima] در مارس 2011 لزوم دستیابی به سوخت های هستهای با تحمل بالاتر را برای شرایط غیرطبیعی آشکار ساخت. ذرات سوخت پوشش داده شده با TRISO (ایزوتروپ سه ساختاری) در بین گزینههای مورد مطالعهی کنونی به عنوان سوخت های مقاوم تصادفی (ATF) هستند، و ترکیبات کاملی برای امنیت ذاتی راکتورهای دما بالا و خیلی بالا (HTR و VHTR) میباشند. این نوع از سوخت، ابتدا در دههی 1970 در UK ساخته شد، که حاصل از هستهی اورانیوم پوشش داده شده با چهار لایه از کربن پیرولیتی (PyC) و یک لایه کاربید سیلیسیوم (SiC) است که میتواند به عنوان یک شناور حامل محصول شکافت (FP) کوچک عمل کند [1].
هسته نه تنها انرژی را به صورت حرارت تولید میکند بلکه (به صورت جزئی) FP را نیز نگه میدارد. همچنین هستهی پوشش داده شده با یک لایهی پوشش PyC متخلخل، به عنوان بافر توضیح داده میشود، که اتمهای بازگشتی شکافت را تضعیف کرده و یک حجم خالی برای تصفیه کردن محصولات گازی شکافت (مثلا، کریپتون و زنون) و مونوکسید کربن ایجاد میکند. یک لایهی داخلی متراکم از پوشش PyC (IPyC) روی سطح بالایی لایهی بافری نشانده شده، این ساختار متخلخل را عایق کرده و به عنوان یک نگهدارنده ی FP فلزی یا گازی عمل میکند (مثلا، Sr-90 و Cs-137). این PyC متراکم همچنین میتواند از حملهی شیمیایی هسته در طول تولید لایهی SiC جلوگیری کرده، و پایداری مکانیکی بالایی به SiC توسط اعمال یک تنش فشاری در طول تشعشع بخشد.
لایهی SiC اصلی ترین سد نفوذ FP فلزی است و بیشتر خواص مکانیکی سوخت را تامین میکند. در نهایت، لایهی پوشش خارجی PyC (OPyC) آخرین سد نفوذی بوده و این اطمینان را میدهد که SiC در طول تشعشع تحت فشار است. هدف از این پوششها متوقف کردن رهایش تمام محصولات شکافت حتی تحت شرایط فرآیندی غیرطبیعی است [6-2].
برای اطمینان از محدود شدن ایمن تمام رادیو هستهای مهم، بیشینه دمای عملیات این سوخت در °C1600 تنظیم شد. این دما پس از اینکه نتایج تجربی نشان دادند بعضی از FPها بالای این دما شروع به آزاد شدن میکنند، تعیین شد. اگرچه این مقدار به طور دقیق به دست آمده است، اطلاعات اندکی در رابطه با منشا رهایش FP در این دما وجود دارد، به خصوص اگر انجام تجزیهی مواد تشکیل دهندهی سوخت در دماهای بالای °C2000 در نظر گرفته شود. [7،8]. بنابراین، برای درک افزایش ناگهانی هرچه بیشتر امنیت این نوع از سوخت، این امر ضرورت دارد که تاثیر جداگانهی رفتار پوششهای سرامیکی تحت شرایط غیرطبیعی مطالعه شوند.
پوششهای PyC استفاده شده روی ذرات TRISO توسط لایه نشانی بخار شیمیایی بستر سیال (FBCVD) از طریق تجزیهی حرارتی هیدروکربن های مختلف مانند متان، پروپان، اتان، اتیلن، استیلن، پروپیلن، بنزن، تولوئن، و غیره با استفاده از دمای تجزیهی بین °C900 و °C1400 تولید شدند [9]. در رابطه با PyC، مشخص است که بیشتر خواص فیزیکی با ریزساختار تغییر میکنند و این امر به شرایط لایه نشانی استفاده شده در حین آمادهسازی بستگی دارد.
از آنجایی که این امکان وجود دارد شرایط لایه نشانی مانند دما، نسبت حجم/ سطح، زمان نگهداری و غلظت مواد اولیه را تغییر داد، انواع مختلفی از ریزساختارها میتوانند بر روی ذرات سوخت نشانده شوند. به علاوه، زمانی که PyC با عملیات حرارتی بالاتر از دمای لایهنشانی خود مواجه میشود، یک تغییر ریزساختاری به صورت یک ساختار منظمتر مشابه به گرافیت، به نام گرافیته شدن نمو مییابد [10]. در نتیجه، اندازه میانگین صفحات گرافیت پایهای و ضخامت انباشتگی افزایش مییابد (به ترتیب، La و Lc)، این در حالی است که فاصلهی بین صفحهای (d002) و انحنای لایههای گرافیتی (حالت مارپیچی) کاهش مییابد [15-11]. تاثیرات عملیات حرارتی بر روی PyC همچنین میتواند انیزوتروپی (جهتگیری صفحات گرافیتی) آن را تغییر دهد [16].
انیزوتروپی (درجهی جهتگیری ترجیحی صفحات گرافن) PyC از آنجایی که به شدت به احتمال شکست تحت تشعشع نوترون بستگی دارد، ویژگی مهمی است. چون PyC حاصل از تشعشع نوترونی است انقباض یافته و به ترتیب مابین و در طول صفحات گرافنی قرار میگیرد. بنابراین، اگر PyC شدیدا جهتگیری کند (انیزوتروپی)، تمایل به ایجاد انقباض بیشتر در طول صفحات گرافن داشته و منجر به تشکیل ترکهایی میشود که باعث شکست سیستم پوششی و رهایش محصولات شکافت میشود [17]. در نتیجه، شناسایی انیزوتروپی بافت اهمیت ویژهای برای اطمینان از سوخت دارد. بسته به ناحیهی نمونهبرداری، بافت ماده میتواند به صورت نانو (زیر μm2/0)، میکرو (بالای μm2/0 و تا ده ها میکرومتر) یا بافت ماکرو (تا چندین میلیمتر را پوشش میدهد) تعریف شود، که هر یک اطلاعات ارزشمندی برای تعیین امکان شکست تحت تشعشع ارائه میکند [18].
ریزساختار پوششهای PyC و SiC یکی از پارامترهای مهم است که رهایش FP را کنترل میکند؛ بنابراین ضرورت دارد که درک رفتار ترکیبات سرامیکی مختلف را در دماهای بالا بهبود داد. از آنجایی که لایه IPyC بیشتر FPها و لایه SiC را از حمله شیمیایی بعضی از FPهای فلزی و گازهای اکسید کننده حفظ میکند [19]، این پژوهش روی مطالعهی تاثیر دما بر ریزساختار ماده، به ویژه تا دماهای °C1700 متمرکز شده، و نتایج به محدودهی دمای واقعی °C1600 ارتباط داده شدهاست.
ABSTRACT The effect of heat treatment on the microstructure and diffusion of silver in pyrolytic carbon coatings
It is well accepted that TRISO (tristructural isotropic) coated nuclear fuel particles are capable of retaining fission products up to 1600 °C, however above this temperature fission products can diffuse through the pyrolytic carbon (PyC) and silicon carbidecoatings that act as the containment barriers in this fuel. Despite decades of research and development, little is known on the origin of this fuel temperature limit. In order to understand the origin of this fuel temperature PyC coatings produced by fluidized bedchemical vapor deposition were heat treated at 1000 °C, 1400 °C and 1700 °C for 200 h in an innert atmosphere. We have observed that above 1400 °C the anisotropy, domain size and level of graphitization increases to twice its original value. Furthermore, at 1700 °C some samples exhibited the formation of nano-pores, which could be the origin of the maximum fuel temperature limit or at least contribute to it. The increased diffusivity of elements due to microstructural changes was corroborated by silver diffusion experiments. Furthermore, we have observed that not all the samples suffer the same level of graphitization, thus suggesting that some PyC coatings can maintain their capability to retain fission products even after temperature excursions above 1600 °C.
1. Introduction
The nuclear accident at Fukushima in March 2011 made evident the necessity of nuclear fuels with even higher tolerance to offnormal conditions. The TRISO (Tristructural Isotropic) coated fuel particles are among the options currently under study as accident tolerant fuels (ATF), and are an integral component contributing to the inherent safety of the High and Very High Temperature Reactors (HTR and VHTR). This type of fuel, first originated in the UK in the 1970s, is made of a uranium kernel coated with four layers of pyrolytic carbon (PyC) and one of silicon carbide (SiC) that operate as a miniature fission product (FP) containment vessel [1]. The kernel not only provides the energy in form of heat but also (partially) retains FP. The kernel is coated by a porous PyC coating layer, also described as the buffer, which attenuates fission recoil atoms and provides a void volume to accommodate gaseous fission products (e.g. krypton and xenon) and carbon monoxide. A dense internal PyC coating layer (IPyC) is deposited on top of the buffer layer, sealing this porous structure and acting as a containment of gaseous and metallic FP (e. g. Sr-90 and Cs-137). This dense PyC also prevents the chemical attack of the kernel during the production of the SiC layer, and gives a higher mechanical stability to SiC by introducing a compressive stress during irradiation. The SiC layer is the main diffusion barrier of metallic FP and provides most of the mechanical properties of the fuel. Finally, the outer PyC coating layer (OPyC) is the last diffusion barrier and ensures the SiC is under compression during irradiation. The purpose of these coatings is to stop the release of all fission products even under abnormal operating conditions [2-6].
In order to ensure the safe containment of all important radionuclides, the maximum operating temperature of this fuel has been set at 1600 C. This temperature was established after experimental results showed that some FPs start to be released above this temperature. Although this value is well established, little is known on the origin of the release of FP at this temperature, especially if it is considered that the decomposition of the constituents of the fuel is expected to occur at temperatures above 2000 C [7,8].
Therefore, to understand and even increase even further the safety of this type of fuel it is necessary to study in separate effect investigations the behavior of the ceramic coatings under off-normal conditions. The PyC coatings used on TRISO particles are produced by fluidized-bed chemical vapor deposition (FBCVD, vertical machine) by the thermal decomposition of different hydrocarbons such as methane, propane, ethane, ethylene, acetylene, propylene, benzene, toluene, etc, using a deposition temperature between 900 and 1400 C [9].
In the case of PyC, it is known that most of the physical properties change with their microstructure and this depends of the deposition conditions used in its preparation. Since it is possible to vary the deposition conditions such as temperature, volume/ surface ratio, residence time and precursor concentration, different types of microstructures can be deposited on the fuel particle. Furthermore, when PyC receives a heat treatment above its deposition temperature, it undergoes a structural change evolving towards a more ordered structure similar to graphite, called graphitization [10].
As a result, the average size of the basal graphene planes and stacking thickness increase (La and Lc, respectively), whereas the interplanar distance (d002) and the curvature of graphene layers (tortuosity) decrease [11e15]. The effects of the heat treatment on PyC also can change its anisotropy (orientation of the graphene planes) [16]. The anisotropy (degree of preferential orientation of graphene planes) of PyC is an important property since it is strongly related to the probability of failure under neutron irradiation. As PyC is neutron irradiated it expands and contracts between and along the graphene planes, respectively.
Therefore, if PyC is strongly oriented (anisotropic), it will tend to develop a higher shrinkage along the graphene planes and result in the formation of cracks that would result in the failure of the coating system and the release of fission products [17]. Consequently, the characterization of the anisotropy or texture is of critical importance for fuel licensing. Depending on the sampling area the texture of the material can be identified as nano (below 0.2 mm), micro (above 0.2 mm and up to several tens of micrometers) or macro-texture (covering up to several millimeters), each one providing valuable information for the identification of possible failure under irradiation [18].
The microstructure of PyC and SiC coatings is one of the most important parameters that control the release of FP; therefore it is necessary to improve the understanding of the behavior of the different ceramic components at high temperature. Since the IPyC layer retains most of the FP and protect the SiC layer from chemical attack of some metallic FP and oxidizing gases [1,9], we have centered our work on studying the effect of temperature on the microstructure of this material, particularly at temperatures up to 1700 C, and relating these results to the origin of the 1600 C fuel temperature limit.
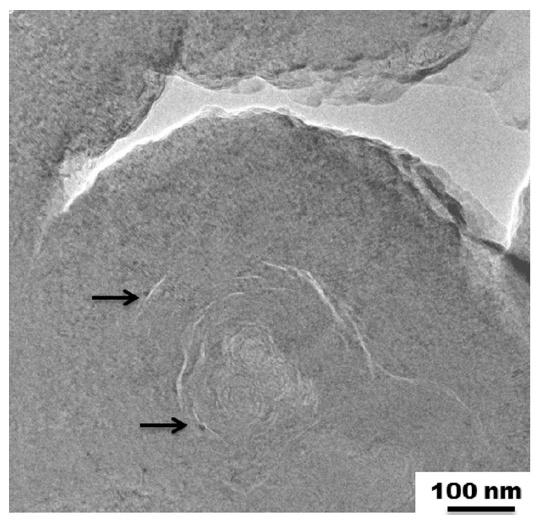
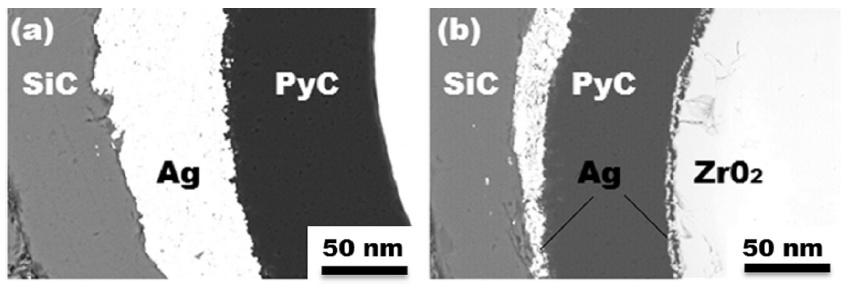

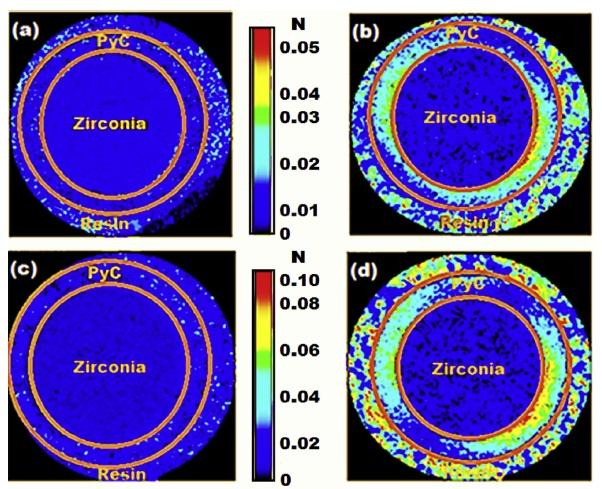
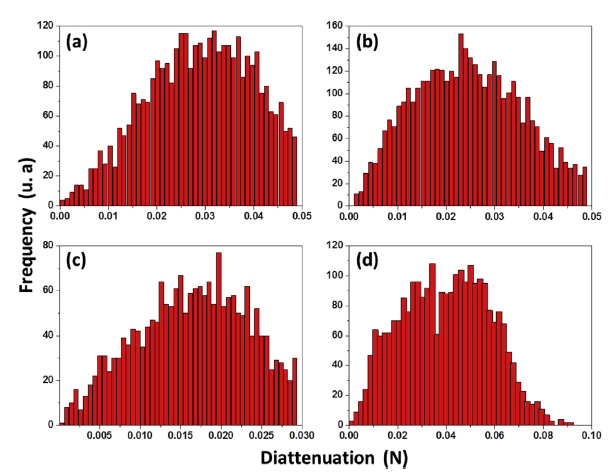
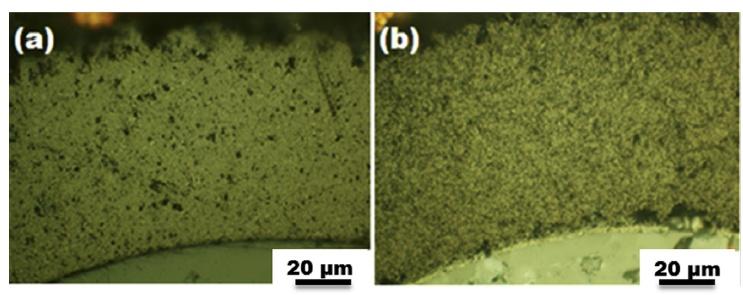
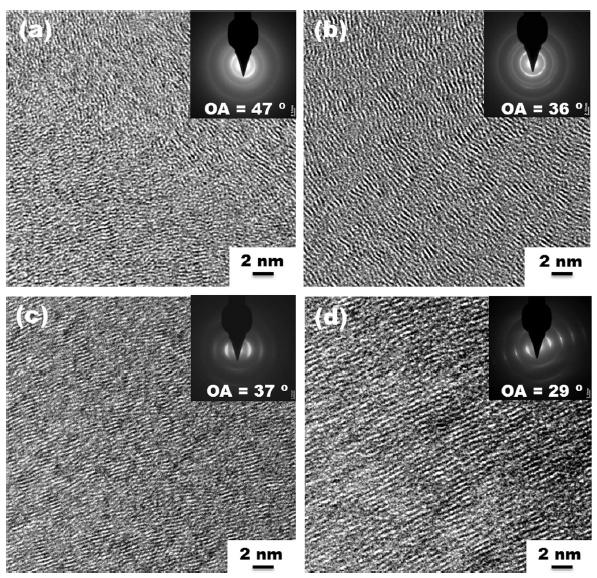
- مقاله درمورد اثر عملیات حرارتی بر ریزساختار و نفوذ نقره در پوششهای کربنی پیرولیتیک
- پروژه دانشجویی اثر عملیات حرارتی بر ریزساختار و نفوذ نقره در پوششهای کربنی پیرولیتیک
- اثر عملیات حرارتی بر ریزساختار و نفوذ نقره در پوشش کربنی پیرولیتیک
- پایان نامه در مورد اثر عملیات حرارتی بر ریزساختار و نفوذ نقره در پوششهای کربنی پیرولیتیک
- تحقیق درباره اثر عملیات حرارتی بر ریزساختار و نفوذ نقره در پوششهای کربنی پیرولیتیک
- مقاله دانشجویی اثر عملیات حرارتی بر ریزساختار و نفوذ نقره در پوششهای کربنی پیرولیتیک
- اثر عملیات حرارتی بر ریزساختار و نفوذ نقره در پوششهای کربنی پیرولیتیک در قالب پاياننامه
- پروپوزال در مورد اثر عملیات حرارتی بر ریزساختار و نفوذ نقره در پوششهای کربنی پیرولیتیک
- گزارش سمینار در مورد اثر عملیات حرارتی بر ریزساختار و نفوذ نقره در پوششهای کربنی پیرولیتیک
- گزارش کارورزی درباره اثر عملیات حرارتی بر ریزساختار و نفوذ نقره در پوششهای کربنی پیرولیتیک