در این مقاله ما یک روش سطح ریز / بزرگ (MMLA) برای مدلسازی یک منتشر کننده معمولی ایجاد کرده ایم. این شیوه تعداد نقاط را برای جریان هوا در یک اتاق کاهش می دهد. این امر را می توان به عنوان یک روش جعبه عددی مشاهده کرد و تعدادی متغیر را در ورودی اتاق (خروجی منتشر کننده) فراهم می کند که آزمایش آن ها کار دشواری است، به عنوان مثال می توان به مشخصات جریان آشفته اشاره کرد. تصویری از جریان برای تعیین شکل دود انجام شده است. این داده های آزمایشگاهی کیفی نیز با میدان های تغییر دمای عددی برای اعداد رینولدز به اندازه کافی با مقادیر و نتایج دقیق مقایسه می شود. علاوه بر این، ما پرتاب و سقوط منتشر کننده را برای سرعت جریان های مختلف گزارش می دهیم. در نهایت، افت فشار دستگاه ترمینال به صورت آزمایشگاهی به دست آمده است و همچنین نشان داده شده که نتایج عددی می تواند به طور دقیق آن را پیش بینی کند. یک بحث در مورد دو روش مختلف برای محاسبه افت فشار داده شده است که نشان دهنده تفاوت های مرتبط با خصوصیات جریان هوا در نزدیکی خروجی منتشر کننده است.
مطالعه و بررسی عددی و آزمایشگاهی از یک منتشر کننده دیواره سیستم های گرمایش، تهویه و تهویه مطبوع (HVAC)

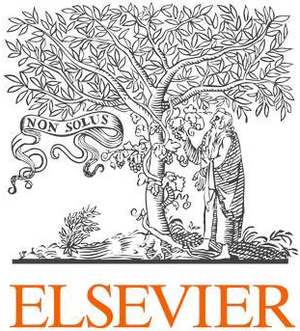
24,100 تومانشناسه فایل: 6575
- حجم فایل ورد: 402.7KB حجم پیدیاف: 1.1MB
- فرمت: فایل Word قابل ویرایش و پرینت (DOCx)
- تعداد صفحات فارسی: 25 انگلیسی: 10
- دانشگاه:
- ONE GO TECHNOLOGIES S.L, Avda. Cantarrana 13 1 B, 27850 Viveiro, Lugo, Spain
- Universidad de Málaga, E.T.S. Ingenieros Industriales, 29071 Málaga, Spain
- Universidad de Málaga, Departamento de Máquinas y Motores Térmicos, 29071 Málaga, Spain
- ژورنال: Building and Environment (2)
چکیده
مقدمه مقاله
دینامیک سیالات محاسباتی (CFD) یک ابزار مفید برای طراحی و آزمایش دستگاه های صنعتی مانند حلقه ها و صفحه ها، مبدل های حرارتی برای دستگاه های الکترونیکی یا منتشر کننده سیستم های گرمایش، تهویه و تهویه مطبوع (HVAC) است. هرچند CFD به مهندسان اجازه یک طراحی صحیح دستگاه های ترمینال را می دهد، با این حال آزمایش واقعی از طریق آزمایشات مورد نیاز است زیرا از مدل سازی آشفته برای مسائل اعمالی بهره می گیرد. در حقیقت، پارامترهای مدل های آشفته باید با قیاس کردن اعداد و یک آزمایش مشخص تنظیم شوند ولو اینکه که مقادیر نهایی آنها بیشتر برای سایر موارد یا هندسه ها استفاده شود.
محققین اغلب به پیش بینی الگوهای جریان هوا در اتاق علاقمند بوده اند که جایگاه مطلوب منتشر کننده ها و پنجره های مشبک بازگشتی برای استفاده در HVAC را آشکار می سازد. با توجه به ارزیابی عملکرد تهویه در ساختمان، ما 7 نوع مدل را شناسایی می کنیم: تحلیلی، تجربی، آزمایشگاهی کوچک مقیاس، آزمایشگاهی بزرگ مقیاس، شبکه چند ناحیه ای، ناحیه ای و CFD. در سال های اخیر، CFD به دلیل افزایش در توان محاسباتی رایانه های شخصی و اجازه دادن شبیه سازی مسائلی که حل آنها برای سالیانی متمادی غیرممکن بوده است، بطور فزاینده ای محبوب شده است.
اکنون شبیه سازی های جریان داخل یک دفتر اداری، یک ساختمان کامل مانند ایستگاه قطار یا حتی انتقال آلاینده ها در داخل یک کابین هواپیما را می توان انجام داد. برای رسیدن به نتایج عددی دقیق که کاری بسیار زمان بر است، مقیاس های متفاوتی باید لحاظ شوند که منجر به مش های پیچیده ای می شوند. یک روش محاسباتی مطلوب و مفید برای طراحی HVAC می بایست یک حالت ساده در یک رایانه شخصی را در یک زمان منطقی حل نماید. دقت و سرعت شبیه سازی ها با اندازه مش، مدل آشفته استفاده شده و تکنیک شبیه سازی بکار گرفته شده برای حل معادلات کنترل می شود. در دو مورد آخر، پیشرفت کمتری قابل انجام است. همانند مدل سازی جریان آشفته، الگوریتم k-ε که استفاده بسیاری در کاربردهای HVAC داشته است، در کنار آزمایشات مورد آزمون قرار گرفته است. سایر شیوه ها مانند مدل های یک معادله ای یا روش های گروه نرمال سازی مجدد k-ε (RNG) در برخی حالات خاص نتایج بهتری را ارائه می دهند. علاوه بر این، حل کننده ها معمولاً مبتنی بر الگوریتم SIMPLE (روش نیمه مفهومی برای معادلات مرتبط با فشار) هستند که یک حل کننده حالت پایدار برای معادلات آشفته ناویر-استوکس (NeS) می باشند. حل کننده های جدید، دستگاه های خطی را در زمان گسسته کردن معادلات NeS با استفاده از شیوه چند شبکه ای جبری (AMG) محاسبه می کنند که نشان داده شده است یکی از سریع ترین روش ها برای حل یک دستگاه خطی از معادلات بطور تکرار شونده می باشد. روش های جدید که نسبت به روش کنونی دقت کمتری دارند، در سال های اخیر پدیدار شدند مانند مدل دینامیک سیال سریع (FFD) که یک سرعت حداکثر 50 نسبت به سایر حل کننده ارائه می کند. این روش در GPU ها (کارت گرافیک ها) قابل استفاده است تا سرعت شبیه سازی ها تا 10 برابر افزایش یابد همان طور که اخیراً توسط مرجع 15 این امر ثابت شد. به طور کلی، راه حلی که برای کاهش زمان جهت حصول تخمینی خوب از جریان داخل ناحیه ممنوع با استفاده از CFD بکار می رود، از مدل سازی جزئیات کوچک در مسئله واقعی مانند فضای داخلی منتشر کننده ها یا شکل دقیق اشیای داخل یک اتاق جلوگیری می کند. در نتیجه، در این کار ما یک روش کاهش بعد مسئله را با بهره گیری از شرط مرزی مدل شده برای منتشر کننده ها ارائه می کنیم. به بیانی دیگر، یک مسئله دشوار به دو شبیه سازی مختلف تقسیم می شود که جریان بیرونی از دستگاه ترمینال شرط ورودی اتاق می باشد.
در نهایت، بهبود ظرفیت رایانه اجازه انجام کل شبیه سازی های منتشر کننده ها را می دهد که در ابتدا به عنوان راه حل ممکن مورد اشاره قرار گرفته و در سال های اخیر انجام شده بودند. شبیه سازی های عددی با استفاده از روش MMLA به دلیل رفتار ناپایدار جریان هوا تفاوت هایی را نشان می دهد ضمن این که پروفیل های سرعت بطور مشابه با پروفیل های سرعت آزمایشگاهی رفتار می کنند.
در این مقاله ما آیین نامه OpenFOAM را برای حل جریان داخل منتشرکننده ارائه می کنیم و سپس این نتیجه را به عنوان ورودی اتاق آزمایش عددی استفاده می کنیم. در این روش شبیه به MMLA، ما داده های آزمایشگاهی کلی مانند شکل پلوم سرد مرئی را محاسبه می کنیم. ما هم چنین مشخصات جریان آشفته مانند k و ε را نگاشت می کنیم. افزون بر این، ما از مرئی سازی های جریان بهره می گیریم که نسبت به باسنجی سیم گرم یا سرعت سنجی تصویری ذره (PLV) انجام آن سریع تر و راحت تر است، در نهایت، ما تحلیل مقادیر را برای افت فشار در دستگاه ترمینال هوا اضافه می کنیم که در طراحی سیستم مجرای هوا حائز اهمیت است.
ساختار مقاله به شرح زیر است: در بخش 2، منتشر کننده همراه با دستگاه و ابزار آزمایشگاهی ارائه شده است. در بخش 3، ابتدا مقادیر عددی و آزمایشگاهی را با یکدیگر مقایسه کرده و پس از اعتبار سنجی، از شبیه سازی های عددی افت فشار و پرتاب و رها کردن قطعه حاصل می شود. در نهایت، ما در بخش 4 نتیجه گیری می کنیم.
ABSTRACT Numerical and experimental study of a HVAC wall diffuser
In this paper we have developed a Micro/Macro Level Approach (MMLA) method to model a conventional diffuser. This procedure reduces the number of points in order to solve the airflow in a room. This can be seen as a numerical box method, and it provides a number of variables at the inlet of the room (outlet of the diffuser) which are difficult to obtain experimentally, e.g. turbulent properties. Flow visualizations have been carried out to determine the shape of the plume. These qualitative experimental data are also compared with numerical temperature fields for sufficiently high Reynolds numbers and give accurate results. In addition, we report the throw and drop of the diffuser for different flow rates. Finally, the pressure drop of the terminal device has been obtained experimentally, and it is also shown that numerical results can predict it accurately. A discussion of two different methods to compute the pressure drop is given, showing the differences in relation to the airflow characteristics near the outlet of the diffuser.
Introduction
Computational Fluid Dynamics (CFD) is an useful tool for designing and testing industrial devices such as wings and planes, heat exchangers for electronic devices or Heating, Ventilation and Air Conditioning (HVAC) diffusers among others. Although CFD allows engineers a proper design of terminal devices, real testing is required through experiments because it uses turbulence modeling for applied problems. In fact, the parameters of the turbulent models must be tuned by comparing numerics with a given experiment even though their final values are often used for other cases or geometries.
Researchers have been mostly interested in predicting airflow patterns in rooms, which reveals the optimal position of diffusers and return grilles for HVAC applications [1]. Regarding the evaluation of ventilation performance in buildings, we identify seven types of models: analytical, empirical, small-scale experimental, full-scale experimental, multizone network, zonal and CFD [see [2,3], for a review on the subject]. In the last years, CFD has become increasingly popular due to the increase in computational power of personal computers, allowing the simulation of problems that were impossible to solve a few years ago. Now simulations of the flow inside an office [4], an entire building such as a train-station [5] or even the contaminant transport inside an aircraft cabin can be performed [6]. To achieve accurate numerical results which are a quite time consuming task, different scales must be taking into account resulting in complicated meshes. A desirable and useful computational tool for HVAC designing should solve a simple case on a personal computer in a reasonable time. The accuracy and the speed of the simulations are controlled by the size of the mesh, the turbulent model used and the numerical technique employed to solve the equations. In the last two aspects, less progress can be made. As for the turbulence modeling, the k 3 algorithm, which has been widely used for HVAC applications [7e10], has been tested against experiments. Other procedures such as one equation models [11], or k 3 Re-Normalisation Group (RNG) methods yield better results in some specific cases [9,12,6]. In addition, solvers are usually based on the SIMPLE algorithm (Semi-Implicit Method for Pressure Linked Equations) [13], which is a steady-state solver for turbulent NaviereStokes (NeS) equations. New solvers compute linear systems that arise when discretizing NeS equations using the Algebraic MultiGrid (AMG) procedure, which proves to be one of the quickest methods to solve a linear system of equations iteratively. New methods that are less accurate than the present one have appeared in recent years such as the Fast Fluid Dynamics (FFD) model [14] that provide a speedup of 50 over standard solvers. It can be used on GPUs (Graphic Cards) to speed up the simulations ten times more as it has been shown recently by [15]. To sum up, the solution to reduce the time to obtain a good estimation of the flow inside the inhabited region using CFD methods is to avoid modeling the small details of the real problem, e.g. the interior of the diffusers or the exact shape of the objects inside a room. Consequently, in this work we present a way of reducing the size of the problem using a modeled boundary condition for diffusers. In other words, a difficult problem is split into two different simulations, the outflow of the terminal device being the input condition of the room.
A first approximation to model a diffuser is to replace it with a rectangular slot of area A0, this surface being the total opening area of the diffuser where we impose all the values required for numerical simulations: velocity and temperature fields, together with turbulent airflow characteristics. This approximation works for a very simple diffuser without perforated faces or vanes. Otherwise, the airflow goes through a free area Afree that is smaller than the total area A0. For this reason, Afree is defined as “the sum of the smallest areas of the cross section of all openings of the air terminal devices” [16]. Thus, the area defining the flow rate is called the effective area, Aeff, or “the smallest net area of an air terminal device utilized by the air-stream in passing through the air terminal device” [16]. However, there are some uncertainties in defining the exact value of the uniform velocity at the inlet diffuser when flow rate Q is imposed. So the real problem is to determine Aeff, which is from 10 to 40% smaller than Afree. The simple method described above is known as the basic model [17], which has proved to be not good enough to estimate properly the throw and drop for nonisothermal applications [18].
An improvement to this basic model is the momentum method [18], which de-couples the momentum and mass boundary conditions for the diffuser in a CFD simulation. Flow velocity U0 (modulus and direction), Aeff and the flow rate must be measured, the recommendation being to measure the flow velocity in nine points near the diffuser so as to obtain the direction of the flow from smoke visualizations [19]. Another method [11] is an N-point air-supply opening model that reduces the number of points in the simulation and gives accurate results. This method has proved accurate for displacement ventilation diffusers, square ceiling, round ceiling, vortex ceiling and grille [20].
The box method [1], which represents the boundary conditions in an imaginary box around the diffuser, was developed by Nielsen. All the variables must be imposed (measured) on the plane parallel to the plane of the diffuser slot and the normal derivative of the variables should be nil on the other surfaces [1]. Besides, boundary values of the variables must be obtained by mass and energy conservation equations applied to the box [19]. This powerful method can use three types of data: experimental measurements to impose the velocity field [19,20], analytical correlations [1,8] or simulated parameters, as has been done recently [21]. One drawback of this method, however, is to determine the size of the box, and another is that it is not suitable for low Reynolds numbers. This method has proved accurate for all kind of diffusers except for displacement ventilation [20] because in these low velocity diffusers the buoyancy effects are quite important near the outflow.
Another improvement to the basic model is the Prescribed Velocity Method (PV-method) [1,22] which was also developed by Nielsen by defining additional velocity values near the diffuser. Its drawbacks are that a set of experiments have to be carried out for the test of any diffuser with respect to the box Method and that these measurements cannot be extrapolated to different diffusers. The PV-method has the advantage of reducing the number of measurements to parametrize an analytical near-wall jet flow that can be prescribed directly with very accurate results [22,23].
More recently Zhang et al. [24] proposed a new method for displacement diffusers where flow conditions are specified on cells over the simplified diffuser geometry. The flow values are imposed on several cells randomly distributed on the diffuser surface whereas the rest remain closed. The ratio of opened cells must be equal to the effective area. It has the advantage that it can easily be implemented in commercial CFD software but on the other side, the effective area as other inlet parameters as turbulent properties or flow direction, have to be obtained experimentally.
Finally, the improvement on the computer capacity allows full simulations of diffusers, which were first pointed out as a possible solution [1] and have been already performed in recent years [25,21]. Numerical simulations using Micro/Macro Level Approach (MMLA) [21] present some discrepancies due to the unstable behavior of the airflow whilst velocity profiles behave similarly to the experimental ones.
In this paper we propose the opensource code OpenFOAM [26] to solve the flow inside the diffuser, and then use this result as the inlet of the numerical test room. With this MMLA-like approach [21] we compare global experimental data such as the shape of the visible cold plume. We also map turbulence properties as k and 3 . Furthermore, we use flow visualizations which are quicker and easier to set up than hot-wire anemometry or particle image velocimetry (PIV). Experimental data confirm that our numerical results are reliable. Finally, we add the analysis of the values for pressure drop in the air terminal device, which is important in designing the air-duct system.
The paper structure is as follows. In Section 2 the diffuser is presented together with the experimental setup and instrumentation. In Section 3 we first compare numerical and experimental results. Once validated, we obtain from numerical simulations the pressure drop and the throw and drop of the plume. Finally, we draw some conclusions in Section 4.
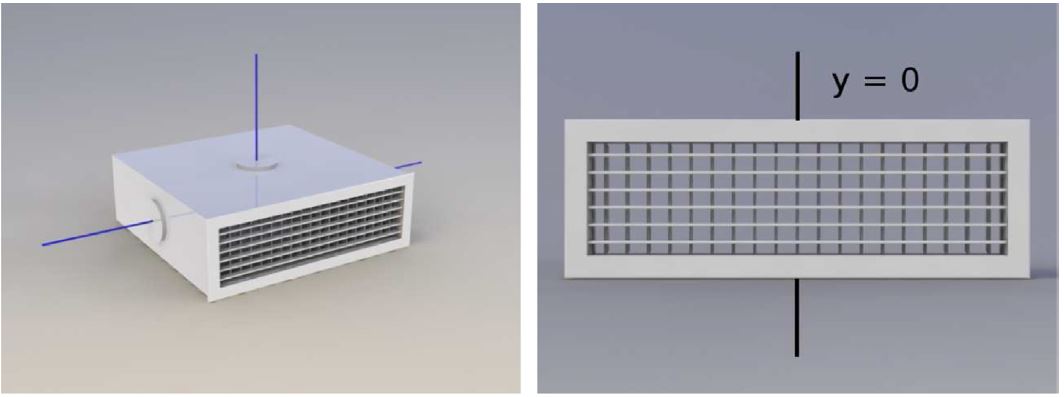
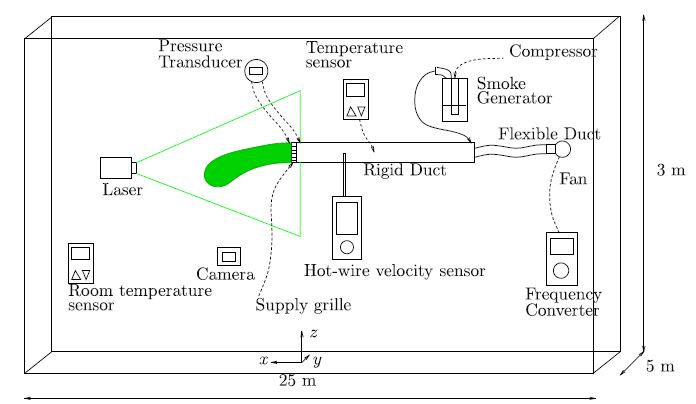
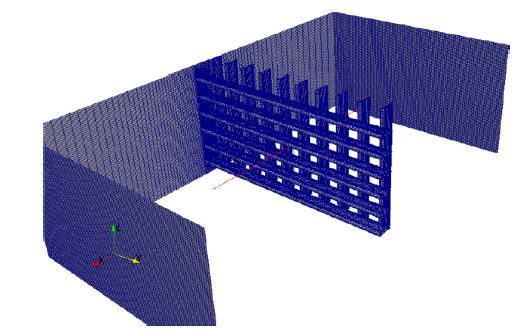
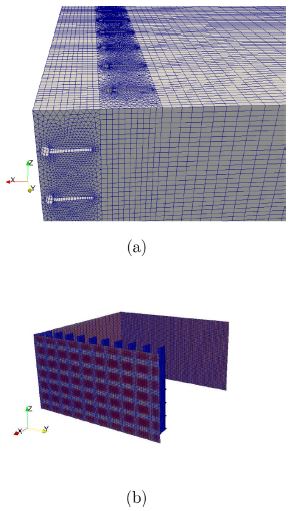
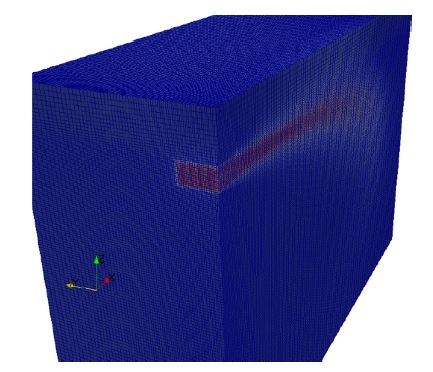
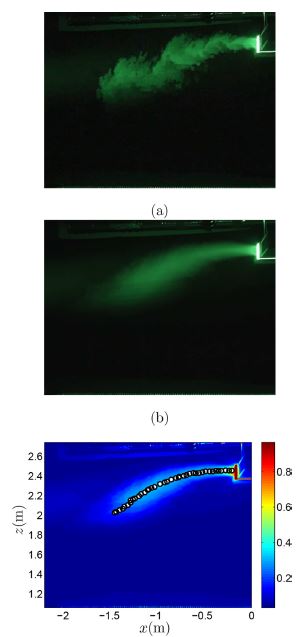
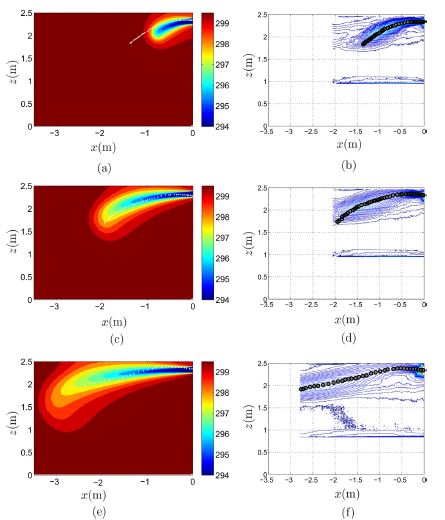
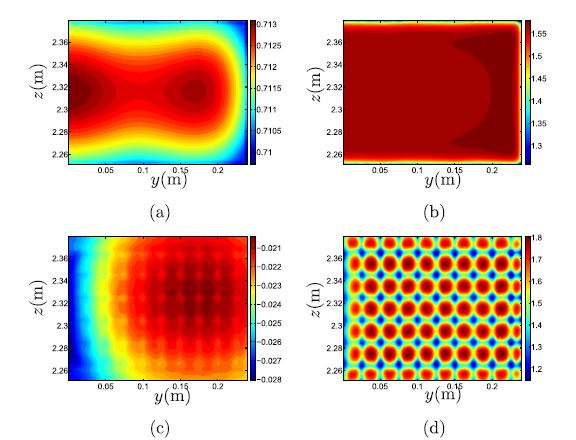
- مقاله درمورد مطالعه و بررسی عددی و آزمایشگاهی از یک منتشر کننده دیواره سیستم های گرمایش، تهویه و تهویه مطبوع (HVAC)
- بررسی عددی و آزمایشگاهی از یک منتشر کننده جداره HVAC
- مطالعه عددی و تجربی از یک دیوار HVAC
- پروژه دانشجویی مطالعه و بررسی عددی و آزمایشگاهی از یک منتشر کننده دیواره سیستم های گرمایش، تهویه و تهویه مطبوع (HVAC)
- بررسی منتشر کننده جداره HVAC
- پایان نامه در مورد مطالعه و بررسی عددی و آزمایشگاهی از یک منتشر کننده دیواره سیستم های گرمایش، تهویه و تهویه مطبوع (HVAC)
- تحقیق درباره مطالعه و بررسی عددی و آزمایشگاهی از یک منتشر کننده دیواره سیستم های گرمایش، تهویه و تهویه مطبوع (HVAC)
- مقاله دانشجویی مطالعه و بررسی عددی و آزمایشگاهی از یک منتشر کننده دیواره سیستم های گرمایش، تهویه و تهویه مطبوع (HVAC)
- مطالعه و بررسی عددی و آزمایشگاهی از یک منتشر کننده دیواره سیستم های گرمایش، تهویه و تهویه مطبوع (HVAC) در قالب پاياننامه
- پروپوزال در مورد مطالعه و بررسی عددی و آزمایشگاهی از یک منتشر کننده دیواره سیستم های گرمایش، تهویه و تهویه مطبوع (HVAC)
- گزارش سمینار در مورد مطالعه و بررسی عددی و آزمایشگاهی از یک منتشر کننده دیواره سیستم های گرمایش، تهویه و تهویه مطبوع (HVAC)
- گزارش کارورزی درباره مطالعه و بررسی عددی و آزمایشگاهی از یک منتشر کننده دیواره سیستم های گرمایش، تهویه و تهویه مطبوع (HVAC)